
Mekhail Anwar, MD, PhD, associate professor in residence in the Dept. of Radiation Oncology, recently received an NIH New Innovator Award. His project, titled INSITE (Implantable Photonic Sensors for Immunoresponse in the Tumor microenvironment), proposes to assess response or resistance to immunotherapy by implanting a fluorescence microscope – the size of a grain of rice – into tumors. Below, Anwar talks about working at the intersection of computer chip technology and cancer therapeutics, innovating for more compassionate care, and the power of mentors.
Q. Congratulations on being named a recipient of an NIH New Innovator Award for “moon-shot”- type, high-risk-high-reward research. You are no stranger to prestigious awards, so how does this one feel? How competitive is it? Was this your first time applying for this award?
Thank you! This award feels particularly great. The NIH New Innovator’s focus on high-risk, high-reward research encourages out of the box, transformative solutions to unsolved problems. That emphasis aligns well with my research program – where we are trying to develop new classes of sensors placed within the body that interface with cells and molecules. This opens up a new paradigm of sensing – from directly within the patient.
Beyond pacemakers and neural prosthesis, we haven’t been able to do this. It’s exciting to envision the possibilities if we can begin to understand how the cellular and molecular milieu in tissue change in response to treatment in real time.
The selection process spans a year with over 700 applications submitted, and about 20% being selected to go to a diverse study panel after an initial review. Awards are selected based on innovation and potential to transform the field of the initial application - and have unusually wide impact across many fields.
I am honored and humbled to be part of this process and the group of awardees. Not unsurprisingly, it required some persistence. This was the second time I applied for this award.
Q. Who is on the project team?
The project team and collaborators embody the multidisciplinary nature of this work. Developing INSITE involves fundamental innovations in engineering, optics and image processing. The team spans both UCSF and UC Berkeley’s Electrical Engineering Department. Currently, we have two very dedicated and brilliant electrical engineering graduate students working developing on our first prototype (Rozhan Rabbini and Micah Roschelle) and new methods of generating 3D real-time images of cells in tissue from our implantable imagers.
But the project is not possible without innovations in the world of electronics and biology.
On the UCSF side, we work closely with Dr. Charles Craik, building on his work to design custom molecules that optically label key immune cells and molecules in vivo. We also work with experts in cancer immunotherapy, such as Dr. Barcellos-Hoff, to translate our imaging results into actionable knowledge of the immune systems response to therapy.
Q. Please talk about your project and the fluorescent imager you developed. What problem is your project addressing?
In caring for cancer patients, I found myself continually confronted by two recurring questions: (1) Why do some patients, ostensibly with the same tumor type – with identical appearances under the microscope, and same cancer stage – respond so differently to the same therapy? We all are familiar with stories of how some patient’s tumors completely resolve and they are cured, while others barely shrink or return quickly. And (2), why do our patients have to wait months to know if a therapy is effective? What prevents our knowing in the hours, or at least days, after therapy is given that it is working?
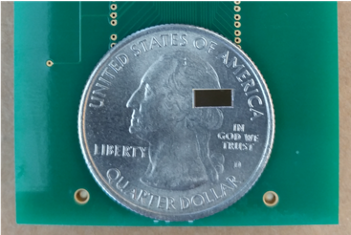
The answer is that we have no method to accurately predict how individual patients will respond to a given treatment. The immense differences in tumor biology, patient-to-patient heterogeneity and complex interactions with the patient’s own body – as well as yet undiscovered processes governing treatment response – make prediction at the individual level extremely hard. This lack of predictability of treatment response is even more consequential with the recent introduction of immune-oncology therapy – a powerful approach that unlocks the body’s own immune system to fight cancer that is fundamentally changing how cancer is treated. Some patients obtain a game changing response. However, less than 50% of patients obtain a durable response to immune-oncology therapy.
Knowledge of instantaneous treatment response would circumvent our inability to predict patient-specific responses, and allow us to quickly pivot away from ineffective treatments to more effective ones, realizing the potential of personalized medicine. Clinical imaging – such as CT, MRI or PET - is a powerful and essential tool in oncology, but is limited to imaging large-scale (centimeter-scale) changes – which can take months to develop.
Ideally, we could take frequent biopsies and visualize tissue under a microscope to gain direct insight into tissue response, but it is impractical to do an invasive procedure repeated basis.
I thought to myself that what we really needed, was a "wireless biopsy." A platform that would allow us to continuously see inside the tumor – at the cellular level. This would enable visualization of cell migration, division and death, in real-time, from deep within tissue. My lab’s prior work has focused on how you build miniature microscopes on a microchip, and so it became clear that we could have a big impact on patient care if we could place these millimeter-scale microscopes inside tumors and have them wirelessly transmit images, watching the tumor microenvironment and immune response evolve in real-time in response to immunotherapy.
Q. How would the microchip be implanted, and at what point in the patient’s course of treatment? How long would it remain?
Implantation would happen through a simple biopsy. INSITE will be designed to be similar in size to a grain-of-rice, and can be either inserted routinely at during a diagnostic biopsy, or a dedicated biopsy. In radiation oncology, we frequently insert "fiducials"– small pieces of gold the size of a grain of rice in the tumor – through a core biopsy needle so that we can quickly image the tumor and precisely locate and "lock onto" it. Right now, these fiducials are passive – and our goal is to turn them into intelligent sensors. Ideally, INSITE would be implanted at diagnosis and monitor tumors throughout their entire course of treatment. INSITE would remain in the patient – at most there may be a thin fibrotic encapsulation that forms around the device, but this would be, in principle, no different than clips left in after surgery.
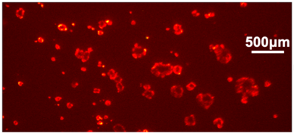
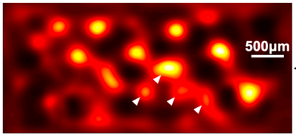
LEFT: An image of a clusters of cells. RIGHT: The image acquired from Dr. Anwar's chip-based fluorescence imager. The arrows identify specific cell clusters and their corresponding image on the sensor.
Q. For people unfamiliar with fluorescence microscopy, can you describe what in vivo, intratumoral images would look like? And how would resistance- or response to therapy be determined?
That’s a great question. Fluorescence microscopy is what allows us to peer deep inside cells and unlock their secrets. It consists of using molecules linked to a (fluorescent) optical tag. This optical tag is a small molecule that is chemically linked to an antibody which binds to the target cell of interest. The optical tag absorbs light at one color and re-emits it at a slightly different color, which is then imaged by a microscope.
By watching this microscope image, we can infer where cells and molecules are in tissue and how they are behaving. Florescence microscopy allows imaging of living cells, and it enables multiplex imaging – i.e. watching multiple cell types at the same time. Both of these attributes are critical to understanding how tissue evolves in response to treatment.
Despite these advantages, fluorescence microscopy, to date, has been restricted to the laboratory and not been deployed in vivo due to the need for a large microscope and the fundamental physical limits of optical probes – as light can only penetrate a few millimeters to a centimeter in tissue.
We solve this problem by developing a fluorescent microscope the size of a grain of rice – and implanting it into a tumor. The images from these microscopes are not as crisp as from a large table-top microscope (as you might expect), but they can identify clusters of cells very rapidly which is what we need. We are currently working on new techniques by modifying the imager design and employing machine-learning algorithms to better deconvolve the image to improve the resolution.
We expect to be able to see multiple cell types moving in 3D in tissue in real time by the end of the project. We are excited to explore early imaging biomarkers of immune response and resistance. This may be as straightforward as looking at a key immune cell infiltrate, but likely will be a more complex picture of pro-immune and immune suppressive cellular activity.
Q. How long have you been envisioning something like this; and which biotech innovations from the past 10 years make your project possible?
I’ve been looking towards a platform to peer inside patients and give us real-time responses ever since I started practicing medicine – about six years ago – and started asking why can’t we know if our patients are responding to treatment in real time. As a clinician this would have an immediate effect on how I prescribed therapy, allowing me to rapidly determine the optimal therapy for each patient.
My project is possible because we are at a unique moment in time, both in both advances in integrated circuit (or “computer chip”) technology and cancer therapeutics. On the technology end, the field of integrated circuits has been powering electronics for decades, but only recently have we begun to deeply integrate photonics (light-guiding structures) with microelectronics at the chip-level. Similarly, wireless technology has significantly advanced allowing information to be transmitted deeper and deeper in the body.
In parallel, we have a growing armamentarium of immune-oncology agents. These agents, if precisely deployed, may enable patients who currently do not respond (colloquially deemed as having "cold" tumors) to be converted to responding or "hot" tumors. These strategies also include the synergistic incorporation of radiation, and perhaps even theranostics.
Q. Why have you decided to use a head and neck cancer model as proof of concept?
INSITE is a platform, applicable to any cancer type. We are using head and neck cancer as a model system because it has three key advantages: (1) the role of immunotherapy in this disease is rapidly evolving, and there is a critical need to know both whom it is working for, and to have a real-time guide to dynamically adjust therapy for those who are not responding. (2) Key sites of disease, such as oral, oropharyngeal and salivary cancers are anatomically accessible for both INSITE implantation, as well as repeated wireless interrogation given their relatively superficial locations. (3) At UCSF, the head and neck team are leading a trial of a preoperative checkpoint inhibitor, giving us an opportunity to evaluate early signatures of immune response.
While head and neck cancer is our first target cancer, INSITE is a platform that can be used with any solid tumor accessible with a biopsy needle. Natural extensions would be breast cancer, and malignancies that have, to date, been refractory to immunotherapy, like pancreas cancer.
We welcome collaborations to deploy this technology into new cancer types and other diseases. In fact, it can be used to monitor any tissue where chronic changes need to be visualized, such as monitoring transplanted organs for rejection, autoimmune diseases, and progression of premalignant lesions to invasive cancers.
Q. What is the timeline for the project; and what are the major milestones? When might you see in-human use?
While the project spans five years, we are hoping to have a prototype in three years. It would likely take another year and a half to manufacture the device for in-human use and start the first trials.
Key milestones will be fabrication of the implantable sensor, establishing an early biomarker of early immune response, and demonstration of proof of concept in a head and neck mouse cancer model. We aim to show that a tumor that poorly responds to immunotherapy can be rapidly detected, and synergistic therapies can be rapidly iterated to convert a “cold” tumor to a “hot” or responding one.
Q. You have education and training in physics, electrical engineering, and medicine. At what ages did your interests in these areas develop? What were your childhood and adolescent hobbies and interests? What are they now?
As a child, I was really fascinated by airplanes – and wanted to be an aeronautical engineer. These days, I think I’ll be happy if I can just sit in a plane again. But my path was really shaped by my parents – who were both professors – who encouraged me to explore. At UC Berkeley, I majored in physics, and loved the problem-solving nature of the field, but yearned for – what was to me - an impactful application. I considered electrical engineering, but was drawn to the impact that new innovations could have in medicine. But instead of switching gears, I combined these fields. At that time, brain-machine interfaces were being heavily studied as there is a natural link between electronics and the nervous system borne out of the success of pacemakers. But I was curious on how to also link electronics to cells and molecules which is still an untapped area – and began working on microchip-scale optical sensors for biological systems.
One of the advantages of the approach to using computer chip technology for studying biological systems is the inherent rapid progress that the computer chip industry has made, and we can leverage many of those advances including smaller size, mass production facilities, and the ability to place complex computation functions on a small chip. One of the interesting aspects to research in this area, is how do you get electronic devices to interface with cells and molecules.
My hobbies growing up centered on sports – and as it turns out, it’s probably not a good idea to play football without helmets and pads, so eventually I changed over to basketball, and continue to play today. I’ve also grown to love exploring new foods. San Francisco is a great place for that, and it has led to a growing fondness for travel – particularly to explore new foods and how to cook them.
Q. With your background, you could have chosen a number of different career paths? Why medicine and oncology?
The chance to materially affect people’s lives and make the interpersonal connections that are truly unique in medicine is what’s been really important in terms of driving me. Fundamentally, I love problem solving and devising creative solutions to access information or be able to do tomorrow what we could not do yesterday.
Cancer remains one of our world’s fundamental challenges, and is multidimensional both in etiology and in treatment approach. I am privileged to work with such dedicated colleagues spanning radiology, surgery, medical oncology and pathology, among others. Our close interactions also give me insight into what these areas can bring in terms of a solution.
But even more humbling is knowing that there is a lot that we don’t know. This makes building tools for discovery, and ultimately control or actuation of the tumor microenvironment, so critical. My hope is that this approach places physicians and scientists directly into patients – giving us a fundamentally new perspective on how disease manifests and responds to treatment.
Taken together, these factors and the ability to make a large impact have driven my passion for both treating cancer today and innovating new solutions for tomorrow.
Q. What advice do you have for researchers with “high-risk, high-reward” ideas in the current funding environment?
Be creative, bold, collaborative and persistent. While traditional funding mechanisms have trending more towards a conservative approach that is de-risked, the high-risk, high-reward mechanisms challenge you to think big, with unusually broad impact. What can you discover or create that will not only solve the problem in front of you, but can change many other fields if successful? Once you have an idea, although the funding climate is continually challenging, we are fortunate here at UCSF to have thoughtful and generous donors who provide funds for getting an idea off the ground. This is incredibly helpful for getting that first “proof-of-life” for your idea. I thank these donors and encourage them to continue to contribute to the discovery that makes improvement in health care possible.
Be collaborative and share your ideas. The community here at UCSF is a powerful force-multiplier, and by synergistically linking different innovations, the impact of your idea may expand far beyond what you initially had thought. Finally, be persistent. Every success is built on many days when you weren’t successful. Learn from those experiences, seek advice, retool and try again.
Q. Who have been your role models (living or dead) and mentors? What is the importance of a mentor?
My role models have been my parents and one of my earliest mentors in oncology, Daphne Haas-Kogan (Chair, Dept. of Radiation Oncology, Dana-Farber Cancer Institute and formerly at UCSF). I’ve been privileged to be supported by parents who encouraged me to explore, find meaning in my work, and problem solve. My early work in oncology was shaped by Daphne – learning how to not only deeply touch people’s lives through the care and compassion we provide, but how to harness that energy and purpose to solve the problems our patients faced in the laboratory.
Two of my earliest research mentors who supported me and provided invaluable advice, scientific input and ideas were Dr. Catherine Park here at UCSF and Dr. Bernhard Boser at UC Berkeley. The three of us collaborated on the earliest versions of the fluorescence imager – developing it to guide precision breast cancer treatment. Their long-standing and continuing mentorship has made it possible to be where I am today.
Deeply engaging mentorship is critical – at every level. I continue to seek out new mentors who are also collaborators, such as Dr. Charles Craik and Dr. Ali Niknejad. Mentors that are committed to your success and willing to provide critical, detailed, and frank feedback, can increase the chances that your ideas come to fruition and open doors to new avenues of innovation.