Program Leaders
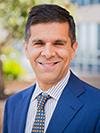
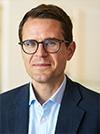
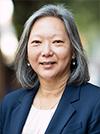
Education and Training Liaison: Nancy Ann Oberheim Bush, MD, PhD
Community Engagement Liaison: Jennie Taylor, MD
The overarching scientific goal of the Neurologic Oncology Program is to use a team approach to advance the understanding of brain tumor biology and drive translation toward more effective and targeted treatments. This is achieved through the close collaboration of multidisciplinary clinicians and scientists devoted to studying brain tumors and combined expertise in genomics, cell signaling, developmental biology, immunotherapy, neuroimaging, epidemiology, and molecular therapeutics.
The Neurologic Oncology Program meets its goal through innovative and impactful research, robust and diverse education and training, and integrated community engagement, all of which are aligned under the following aims:
- Aim 1: To improve understanding of the underlying biology of brain tumors
- Aim 2: To predict patient disease, response, and survival in brain cancers
- Aim 3: To improve therapy for brain tumors
Roster of Neurologic Oncology Program
For details on membership criteria, please see our membership page.